Compendial waters must meet relatively low bacterial content (bioburden) targets. What’s more, pharmaceutical manufacturers must prove that their purified water production systems consistently meet or exceed these performance objectives. Non-compliance with bioburden limits typically carries very high risks for human health and a very high cost for the pharmaceutical manufacturer. For this reason, the sanitization strategies selected for the production of purified water are of utmost importance.
There are a number of effective strategies for controlling bacterial growth in purified water production systems. Many clients choose to combine a number of technical alternatives with strict maintenance schedules to ensure compliance. Other factors to consider are capital cost, system downtime, familiarity with technology and processes, chemical handling risks and labour requirements.
An Overview of Effective Technologies for Disinfection
Une multitude de technologies peuvent être utilisées dans le cadre d’une stratégie globale de désinfection. Étant donné que ces technologies sont généralement utilisées en combinaison les unes avec les autres, nous explorerons les combinaisons courantes dans la section suivante. Cette section présentera simplement chaque technologie, en expliquant brièvement son fonctionnement.
Ozone
Ozone is a powerful oxidizing agent that, when dissolved in water, allows for rapid and highly effective disinfection.
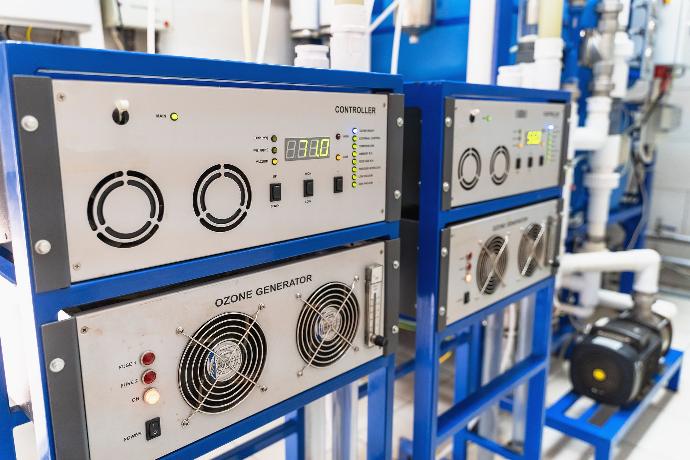
Since ozone is a strong oxidant, systems treated with ozone must be designed with ozone-resistant materials such as 316 stainless steel and fluoropolymers. Furthermore, care must be taken to prevent ozone from coming in contact with any oxidation-sensitive components. Reverse osmosis membranes and ion exchange resins are two ubiquitous parts of the treatment train that need to be adequately protected. Ozone must also be effectively removed from the purified water prior to the point of use to meet compendial requirements.
Ultraviolet Radiation (UV)
UV technology can provide both disinfection and, if so designed, destruction of organic molecules in water. UV is very popular in pharmaceutical applications for its ease of use, its effectiveness and the fact that it adds no substances.
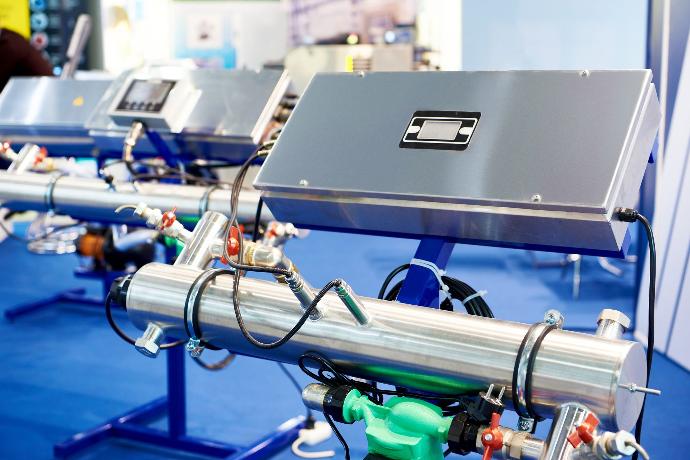
Sub-micron Cartridge Filtration
Typically used for “final filtration”, sub-micron cartridge filters with an absolute pore size of 0.1 or 0.2 microns are another very common disinfection method used in purified water systems. These filters are typically manufactured with polysulfone, polyethersulfone or fluoropolymer membranes.
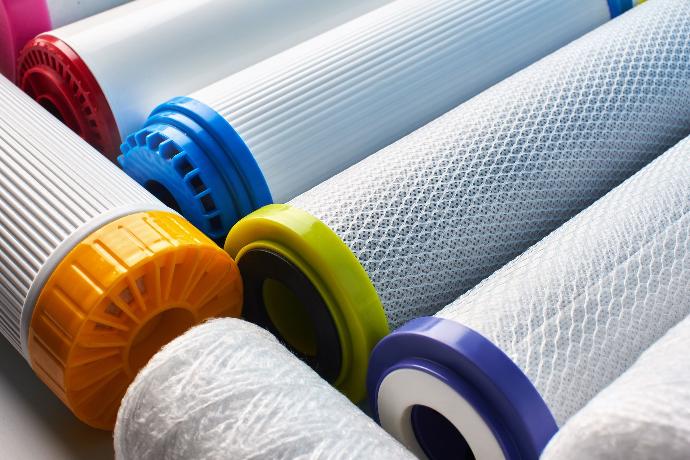
Heat
Hot water, typically between 70-90°C, can be circulated through heat-resistant system components periodically. This hot water sterilization is typically achieved with a heat exchanger built into the purified water system with steam being provided by the plant. Electric water heaters are sometimes used and are typically slower to heat the circulating water, protecting components sensitive to thermal shock but requiring more downtime.
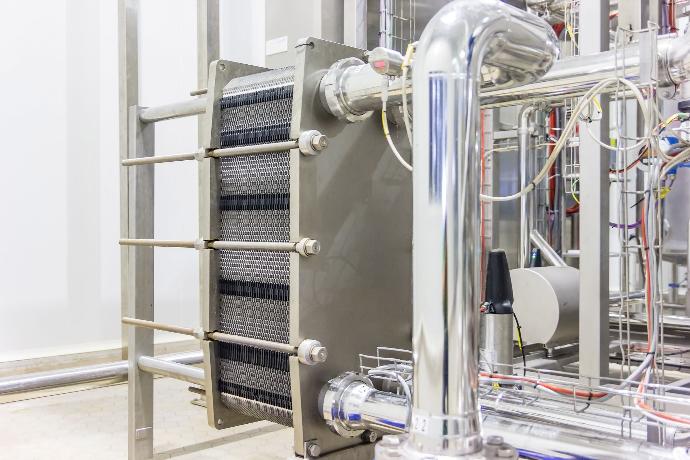
Chemical Sanitization
Various biocides can be used to periodically sanitize purified water systems. In general, the use of these biocides requires that the system be shut down until all traces of biocide have been flushed from the system at the end of the disinfection process.
Great care should be taken when selecting biocides so as to avoid damage to the system components while maximizing the effectiveness of the sanitization process.
Examples of typical biocides used for disinfection of pharmaceutical water systems are peracetic acid, hydrogen peroxide and sodium hypochlorite.
What Doesn’t Work: Emphasizing Bioburden Control Upstream of Reverse Osmosis
When studying ways to control bacterial growth in purified water systems, it’s important to understand what doesn’t work. While this is quite controversial, we have found that efforts to control bacteria upstream of reverse osmosis systems are generally wasted.
RO membranes are a significantly tighter physical barrier than sterilizing grade 0.1 micron cartridge filters and ultra-filtration modules. Nonetheless, bacteria have been shown to inevitably “grow through” these physical barriers. While the exact mechanism behind this phenomenon is still poorly understood, it’s safe to say that some bacteria will eventually make it to the “clean side” of an RO membrane3.
So… that means that we should avoid letting bacteria even get to the RO membrane, right?
While that’s a good idea in theory, the reality is that some bacteria will make it through UV sterilizers. Even if they remove 99.98% of bacteria, that’s still 200 out of every million that will make it to the membrane and potentially colonize its surface. Once that happens, bacterial growth is happening after the UV. As usual, these colonies will grow exponentially until food becomes scarce. Nutrient scarcity doesn’t typically happen on the concentrate side of an RO membrane as new nutrients are constantly flowing in with new feedwater.
In essence, controlling bioburden upstream of an RO can help to delay biological growth on the membranes, but it won’t stop it long-term. It can be used as part of a strategy to lengthen RO run times between cleanings and sanitizations, but it’s not going to effectively keep the RO permeate free of bacteria.
Even if one did manage to prevent any bacteria from crossing the RO membranes, they’d also have to deal with exposure to air during maintenance operations of downstream components like UVs and final filters.
Here are some commonly seen, sometimes costly and generally ineffective ways we’ve seen clients attempt to control bacterial growth:
- UV sterilization upstream of the RO
- Steam sanitization of theactivated carbon filter
- Utilisation de SMBS (sodium métabisulfite) au lieu de charbon actif pour empêcher la croissance bactérienne dans le lit de charbon
- Maintaining a chlorine residual through pre-filtration steps and removing it immediately upstream of the RO
- Heat and/or chemical sanitization of RO pre-treatment components
Effective Bioburden Control Strategies
Now that we’ve seen what isn’t particularly effective, let’s dive into the methods that do work.
UV and Cartridge Filtration As Final Sterilization
By far the most common solution for keeping bacterial counts low is to place a UV sterilizer and absolute 0.1 or 0.2 micron filters at the outlet of the distribution pumps. The UV sterilizer typically kills 99.9% of bacteria while the final cartridge filter provides a physical barrier bacteria cannot pass through. Although sterilizing grade filters provide 100% bioburden reduction for all targeted organisms, biological growth is still inevitably observed downstream of the filters after some time. For this reason, purified water systems also require either periodic or constant disinfection to provide water with acceptably low bacterial counts.
Constant Ozone Disinfection of the Purified Water Storage Tank
A highly effective disinfectant, ozone effectively kills bacteria, penetrates biofilm and oxidizes organic species. Ozone is growing in popularity in pharmaceutical purified water systems and is often used to keep the purified water storage tank sterilized at all times.
Low doses of ozone are used and residuals as low as 20 parts per billion have been shown to be effective in preventing biological growth in purified water storage tanks. As the ozonated water is pumped toward the distribution loop, the ozone sterilizer easily destroys the small amount of residual ozone, much like the UV light from the sun reacts with the ozone layer.
Care must be taken to ensure that all materials that may come into contact with ozone are ozone-resistant. Generally this means changes to the tank vent filter and all gaskets, valves and seals from the tank and to the UV sterilizer.
Periodic Loop Sterilization With Ozone
Systems already equipped with an ozonation system for the purified water storage tank can easily be automated to provide periodic disinfection of the distribution loop. In order for this option to be viable, the distribution piping and components including filters and instrumentation must be resistant to ozone. In most cases, this means 316 stainless steel piping, ozone-resistant elastomers for gaskets, seals and valve seats, and Halar® membrane final filters.
Ozone disinfection of the distribution loop is commonly programmed for a short 15 to 30 minute daily sterilization cycle. During this cycle ozone production is increased and the UV sterilizer is turned off to allow ozone to flow through distribution piping. At the end of the cycle, ozone generation is down-regulated to its standard operating level and the UV sterilizer is switched back on to destroy ozone coming out of the purified water tank.
While this disinfection strategy is extremely effective, some clients decide not to apply it simply due to the higher capital cost of including ozone generation in the system and ozone-proofing the entire distribution loop.
Periodic Disinfection with Other Chemical Biocides
Disinfection with chemical biocides is one of the most common methods in the pharmaceutical, cosmetics and food & beverage industries. We won’t discuss the full gamut of biocides available on the market here. The ones we see most commonly used are made up of peracetic acid and hydrogen peroxide.
While chemical disinfection is effective, care must be taken not to let bacterial growth get out of control. Cleaning becomes significantly more difficult once biofilm starts to accumulate to any great degree within the distribution equipment and piping.
For this reason we recommend that our clients
choosing this strategy carry out chemical SIPs on a frequent and regular
schedule. Most plants see no issues when sanitizations are carried out monthly
or quarterly.
Many
clients find, after having installed and validated their system and maintenance
procedures, that these frequent shutdowns for disinfection are hard to live
with. They require qualified
personnel and the better part of a full work shift to be completed properly.
Periodic Heated Sanitization of the Distribution Tank and Loop
Heated sanitization is one of the most time-tested methods for disinfection in the pharmaceutical industry. Aside from bacteria in the Bacillus genus, no other known bacteria can survive in purified water kept between 70ºC and 90ºC2.
With that said, the periodicity of hot disinfection has a significant impact on its success in purified water distribution systems. If bacterial growth is allowed long enough for biofilms to form at ambient temperatures, standard hot sanitizations may be ineffective since biofilms become harder to remove as they grow thicker. In such situations, it may be necessary to resort to longer, hotter sterilizations and/or chemical disinfection (shock treatment).
Constant Heated Sanitization of the Distribution Tank and Loop
One effective method for preventing any significant biological growth in purified water systems is to simply maintain water temperatures above 70ºC in the entire distribution system. The practicality of this approach depends on a number of factors:
- The length of the distribution loop (cost is much higher for insulated piping).
- The temperature required for the pharmaceutical processes using purified water. Maintaining hot water only to cool it may prove too costly and may increase the risk of bacterial contamination at or after the cooling equipment.
- The energy cost, both economic and environmental, may be considered too high to be acceptable.
That said, a client requiring hot process water can rest assured that their purified water loop will not allow bacterial growth if high temperatures are effectively maintained. Even thermophiles and hyperthermophiles pose a very limited risk with only the Bacillus genus having even an extremely limited potential for growth2 in these conditions.
Biological Growth Prevention by TOC Reduction
The pharmacopeia provides only limited guidance on total organic carbon content in purified water. Here, the pharmaceutical industry could stand to learn from the microelectronics industry.
Microelectronics manufacturing demands a greater degree of purity than pharmaceutical water. While a full microelectronics grade purification system could be considered overkill for pharmaceutical purposes, we can apply some of their learnings to controlling bacterial proliferation in pharmaceutical applications.
Microelectronics manufacturers do not use ozone or high temperatures and they do not typically shut down their water purification systems to perform chemical sanitizations. In their highly competitive industry, they’ve had to devise ways to maximize uptime without compromising quality. How, then, do they keep bacterial growth low enough to prevent quality problems?
The answer is quite simple. They make sure that bacteria have practically no nutrients with which to grow. Extreme TOC reduction is practiced in the microelectronics industry, where TOC limits are now frequently set in the parts per trillion range. Even decades-old plants operate with TOC limits in the single digit parts per billion range, typically around 2 or 3 ppb. Compare this with the typical “below 500 ppb” recommendation seen in pharmaceutical applications.
This approach is far from commonplace in pharmaceutical applications, but it is neither without merit nor forbidden by the pharmacopeia. How the microelectronics industry achieves such high purity is a discussion for another article, but the curious among you can learn more here in the meantime.
Conclusion
Controlling bacterial growth is generally regarded as one of the most important factors in designing pharmaceutical purified water systems and their associated maintenance protocols. While some approaches are tried and true, new approaches based on newer technologies are proving to be equally effective. In some cases, these new approaches may allow you to reduce both costs and business risk.
What are your preferred methods for controlling biological growth in pharmaceutical purified water systems? Leave us a comment below and join the conversation!
Sources
- Gorsky, I. “Pharmaceutical Ultrapure Water Systems – What Pharma Can Learn From Other Industries?”, ValSource and Parenteral Drug Association, https://www.pda.org/docs/default-source/website-document-library/chapters/presentations/metro/pharmaceutical-ultrapure-water-systems—what-pharma-can-learn.pdf. Accessed 22 June 2021.
- Martinez, J. E. “Hyperthermophilic Microorganisms and USP Hot Water Systems.” Pharmaceutical Technology, Feb. 2004, https://cdn.sanity.io/files/0vv8moc6/pharmtech/ee424df517845f9db443bd69ff98fb96b9ce7a0f.pdf. Accessed 22 June 2021.
- Park, S.-K., Hu, J. “Assessment of the extent of bacterial growth in reverse osmosis system for improving drinking water quality.” Journal of Environmental Science and Health Part A Toxic/Hazardous Substances & Environmental Engineering, Jun. 2010, 45(8):968-77.
- Sandle, T. The Problem of Biofilms and Pharmaceutical Water Systems. American Pharmaceutical Review, Dec. 2017, https://www.americanpharmaceuticalreview.com/Featured-Articles/345440-The-Problem-of-Biofilms-and-Pharmaceutical-Water-Systems/. Accessed 22 June 2021.